The future of plant breeding
People began to domesticate wild plants more than 10,000 years ago. Since then, we have been breeding crops in order to make them more resistant and to increase yields. New techniques now make it possible to modify genetic material precisely, which raises questions that our society must discuss and try to answer.
This year’s wheat harvest in the Canton of Zurich was poor – harvest losses were between 20% and 40%. [1] This was due to the wet months of May and June, which encouraged fungal growth. As chemical pesticides can be used only to a limited extent, it is likely Switzerland will have to buy more wheat from other countries than in previous years if it is to meet its needs.
This example shows on a small scale the challenges that face global agriculture: population growth, rising demand for food, limited arable land and climate change. About 90% of global food and animal feed is produced from the cultivation of only ten crop plants, with cereals such as rice, wheat and corn having the greatest contribution. Should unexpected pests or drought hit the cultivation of our crops, famine may result.
Demand for resistant varieties
To secure the global food supply of the future, we need new plant varieties that provide high yields even under changing climatic conditions and which require less use of pesticides. An important alternative to the use of chemical pesticides is to increase the resistance of plants to pests and diseases. Breeders use a diverse range of plant defence and resistance mechanisms, which plants have developed over millions of years of co-evolution with harmful organisms. Improved resistance properties can be achieved through conventional phenotype- and genome-based breeding, or via genetic engineering. Possible improvements by breeding are limited by the inherent characteristics (i.e. gene pools) of the existing varieties.
Targeted genome modifications in plants
In recent years, molecular biology techniques have been developed that modify the genome of higher organisms in a targeted manner (genome editing). [2] Put simply, this is accomplished through two molecules that work together: a recognition module that finds the target site in the genome precisely, and an enzyme that cuts open the DNA at the target site. The target sequences can then be modified, replaced or removed using the cell’s own DNA repair mechanisms. The spectrum of applications ranges from single cells to complete organisms, and from basic research to gene therapy in humans and animals and to plant breeding. In the latter, researchers can introduce new features into crop plants more efficiently by generating desired mutations with great specificity. Of particular interest to research is the possibility of reliable complete inactivation of specific genes; for example, to determine their function.
The specificity for a particular gene, i.e. the precision of the recognition module, comes from a specific molecule that is introduced in the cell and recognises the target sequence exactly. In the early techniques, these were zinc-finger or TALEN proteins, where the recognition code can be adapted to the desired DNA target sequence. Now, however, the technique with the awkward name of CRISPR/Cas is seen as having the greatest potential. This easy-to-use method is also suitable for improving resistance properties and thus increasing crop yields.
From bacterial defence mechanisms to breeding tool
In CRISPR/Cas, a specially structured RNA molecule is used together with an enzyme to recognise a specific point in the genome and cut it open. The desired mutations are then formed during the subsequent cellular repair of the site. CRISPR/Cas works naturally in bacteria as a defence mechanism against invasive DNA (e.g. viruses), but it can be used in cells of all organisms. Various computer programs and gene databases are available to select the appropriate target sequences, in order to prevent unwanted effects on other gene loci or to determine appropriate sequences for the simultaneous modification of various genes. The number of unwanted mutations in other DNA sequences can be minimised to zero with the techniques used today.
Making crops more resistant
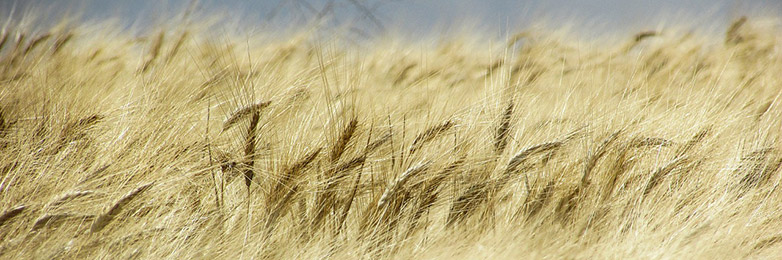
This type of targeted genome modification (mutagenesis) in plants is still at an initial stage of application-oriented technique optimisation. Nevertheless, since 2013 a range of new features have been produced in crop plants (wheat, millet, corn, rice, tomatoes) through genome editing that would have been very difficult and time consuming to achieve with conventional breeding methods. [3] Crop plant genomes in particular are a special challenge since many of them are polyploids, which means they contain more than two copies of their genome – wheat, a hybrid of three wild varieties, even has six.
Similar to humans and animals, plants also use a type of immune system to defend themselves against pathogens such as fungi, viruses and bacteria. As a general rule, specific plant proteins recognise the pathogens and trigger a defence strategy. However, pathogens also use the host’s proteins to make the infection more effective. Increased plant resistance can therefore be achieved either by strengthening the active defence or through the removal of the genes used by the pathogen. The latter can be implemented with CRISPR/Cas through targeted point mutation of the corresponding genes; for example, it is possible to prevent mildew disease in wheat by turning off the six copies of the MLO gene simultaneously. The MLO gene in plants facilitates infection of cereals by mildews (Blumeria graminis f. sp. Tritici) by weakening the plant’s immune response. [4]
Open questions
The special feature of CRISPR/Cas is that the modifications produced in the genome do not differ from naturally occurring mutations in plants and animals caused by environmental influences on the genome, such as natural radioactive radiation, reactive metabolites or even by defects in DNA replication and inheritance. Random mutagenesis by chemical treatment or irradiation has been used in mutation breeding for many years and contributed to major yield gains in our crops in the 20th century. Worldwide this type of mutation breeding led to currently more than 3088 varieties from 190 species.
As plants modified with CRISPR/Cas cannot be distinguished from those modified with conventional breeding techniques, the question arises: if a new breeding method triggers a targeted modification in the genome of the given species that can also be achieved through conventional breeding – admittedly with significantly greater effort – or accidental mutation, should the resulting product be regarded as a GMO (genetically modified organism) or not? The current debate focuses accordingly on whether a regulation should be process- or product-related.
We will look at the question of risk assessment and regulation in greater detail in our next blog post.
A Zukunftsblog series on new plant-breeding techniques
New methods in molecular biology (keyword genome editing) have the potential to make agricultural crop breeding more efficient. This entails socially relevant issues and challenges, such as safety assessments and regulations. The Zukunftsblog is beginning an intermittent series that considers the opportunities and risks opened up by these new breeding techniques.
Johannes Fütterer co-authored this article with external page Manuela Dahinden, Bruno Studer and Wilhelm Gruissem.
Further information
[1] external page Article in the NZZ
[2] The Swiss Academy of Sciences put together a external page Factsheet that provides a good overview and description of selected new breeding techniques.
[3] Song et al. CRISPR/Cas9: A powerful tool for crop genome editing. The Crop Journal 4 (2), 75-82 (2016) external page http://dx.doi.org/10.1016/j.cj.2015.12.002
[4] Wang et al. Simultaneous editing of three homoeoalleles in hexaploid bread wheat confers heritable resistance to powdery mildew. Nature Biotechnol. 32, 947-951 (2014) doi: 10.1038/nbt.2969