Unexpected twist in a quantum system
Physicists at ETH Zurich have observed a surprising twist in a quantum system caused by the interplay between energy dissipation and coherent quantum dynamics. To explain it, they found a concrete analogy to mechanics.
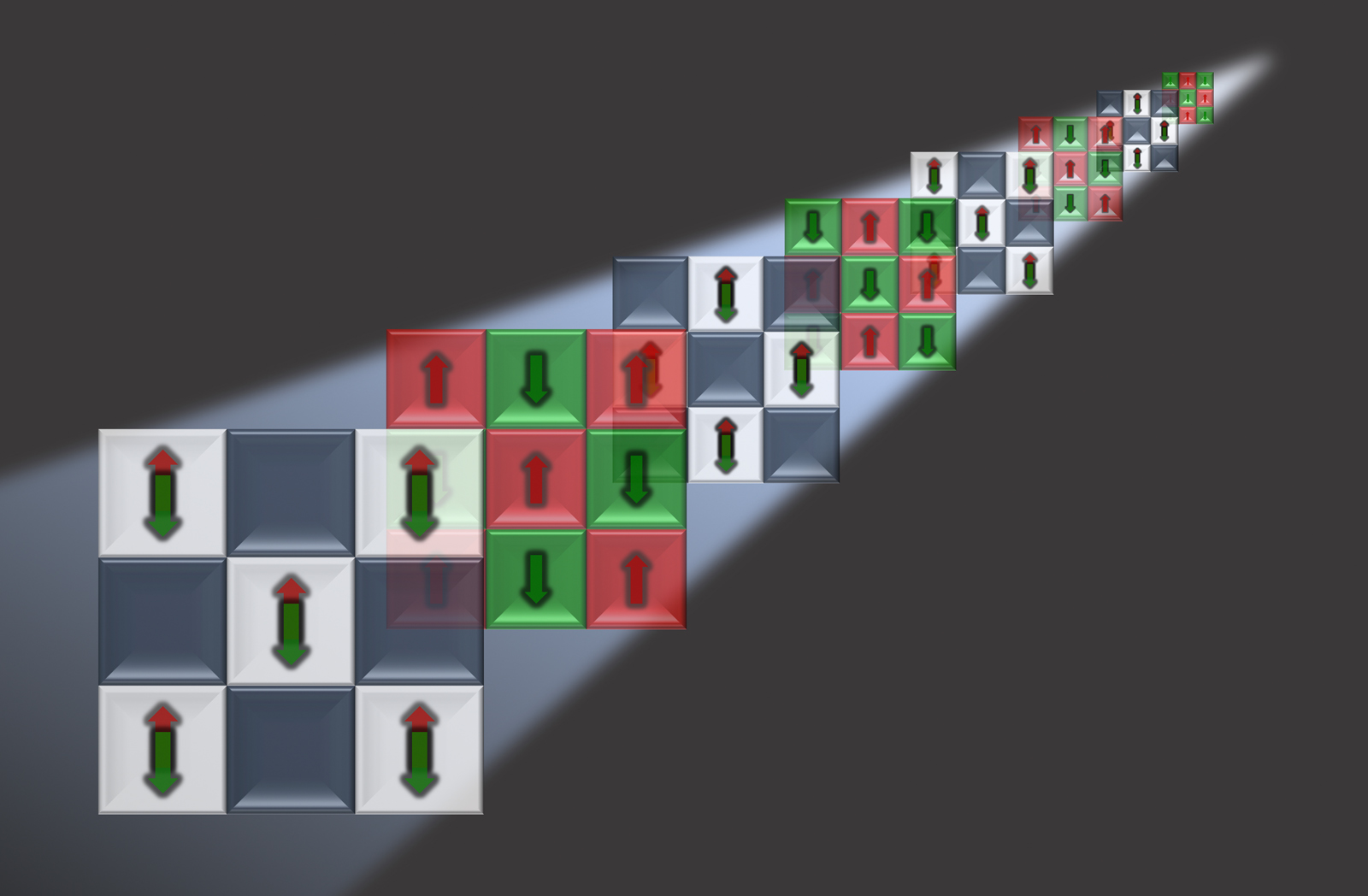
“No scientist thinks in formulae”, Albert Einstein allegedly once told his colleague Leopold Infeld. In fact, especially for physicists, who deal with such abstract things as quantum physics, it is often immensely useful to work with concrete images rather than mathematical symbols. A team of researchers led by Tilman Esslinger, professor at the Institute for Quantum Electronics at ETH Zurich, experienced this when they recently discovered a new effect in their quantum mechanical system. Although they were studying tiny atoms and light particles in their experiment, they were able to understand their observations better through a catchy image: a shaft turning inside a bearing. Their results have recently been published in the journal Science.
A complex quantum system
“We hadn’t really been looking for that effect at all”, says Esslinger. “Only with hindsight did we understand what our data mean.” He and his co-workers had tackled a very complex topic: a quantum system in which the individual particles interact strongly with one another and that is simultaneously driven from the outside and also dissipative. “Dissipative” means that the quantum states of the particles do not just evolve coherently in time – that is, such that their superposition states remain intact. Rather, a controlled connection to the outside world causes the superposition states to disappear little by little. If the dissipation is very strong, they disappear very fast, and as a result the particles then behave almost like in classical physics, which we know from everyday experience. Without any dissipation, on the other hand, the way the particle system evolves in time is dictated purely by quantum mechanics – an ideal case that is used by physicists to build quantum computers, for instance.
Atomic patterns
“Those two extremes can be calculated and understood quite well”, explains Tobias Donner, who works as Senior Scientist in Esslinger’s lab. “By contrast, it’s much more difficult to deal with systems in the middle, where coherent evolution and dissipation are equally important.” To build such a quantum system in the laboratory, the physicists cooled atoms down to temperatures close to the absolute zero of around -273 degrees Celsius and exposed them to a focussed laser beam that traps and drives the atoms inside a kind of lattice made of light. Each atom also has a “spin” that can point up or down (much like a compass needle that points to the North or South). On top of that, the cold atoms are surrounded inside a cavity by two mirrors that reflect the light scattered by the atoms back and forth.
The interplay between the atoms, the laser beam and the light in the cavity now causes the atoms to arrange themselves spontaneously in a checkerboard pattern. This can happen in two different ways. In one of them, there are atoms only on the “white” squares, as it were, whereas the black squares remain empty (see figure). In the other case there are also two types of squares, red and green, but now the red squares are only occupied by atoms whose spins point up, whereas on the green squares there are only atoms whose spins point down.
Surprising twist
Which of the two alternatives the atoms prefer depends on the direction of oscillation of the laser beam that irradiates them, strictly according to the rules of quantum mechanics – at least, that is, if the atoms are not exposed to any dissipation. When the physicists carried out the experiment in a regime where the influence of dissipation (caused by a loss of photons from the cavity) was large enough, something unusual happened. “Our data no longer showed us one of the two patterns, but rather it seemed as though the atoms were turning through the patterns over and over, with a particular sense of rotation”, Esslinger describes the unexpected results. “That was an exciting discovery – but we had absolutely no idea why it was happening.”
An unusual force
By simplifying the quantum mechanical equations describing their experiment, the physicists were eventually able to discover an analogy to a mechanical system. In fact, the formulae bore a striking resemblance to those describing a shaft turning inside a bearing. Between the shaft and the bearing, there is a viscous lubricant that is supposed to ensure a uniform rotation. However, if the shaft moves away slightly from the centre of the bearing, a rather unusual kind of friction force arises that depends on the position of the shaft. The force comes about because in one direction the distance between the rotating shaft and the stationary bearing is reduced, and hence different friction forces act on the shaft and the bearing. The resulting position-dependent force is perpendicular to the direction in which the shaft has moved. As a consequence, the centre of the shaft starts spiralling around the centre of the bearing.
Now that the physicists are able to describe the unexpected quantum effect with a concrete image, they are already thinking about the next step: to exploit it in order to deliberately steer and control quantum systems. “Normally, dissipation alters or weakens existing quantum effects – but here we have an effect that actually owes its existence to dissipation”, says Esslinger. Whether similar effects could possibly be more widespread in quantum systems, and how they might be used in the quantum technologies that are currently being developed, are therefore questions that are now on the researchers’ minds.
Reference
Dogra N, Landini M, Kroeger K, Hruby L, Donner T, Esslinger T: Dissipation-induced structural instability and chiral dynamics in a quantum gas. Science, Vol. 366, Issue 6472, pp. 1496-1499 (2019) DOI: external page 10.1126/science.aaw4465