A contradiction at the heart of physics
Quantum mechanics describes the forces that hold the world together on the smallest scale. The theory of relativity explains the world at the cosmic level. The two seem incompatible – and a unifying theory is nowhere in sight.
- Read
- Number of comments
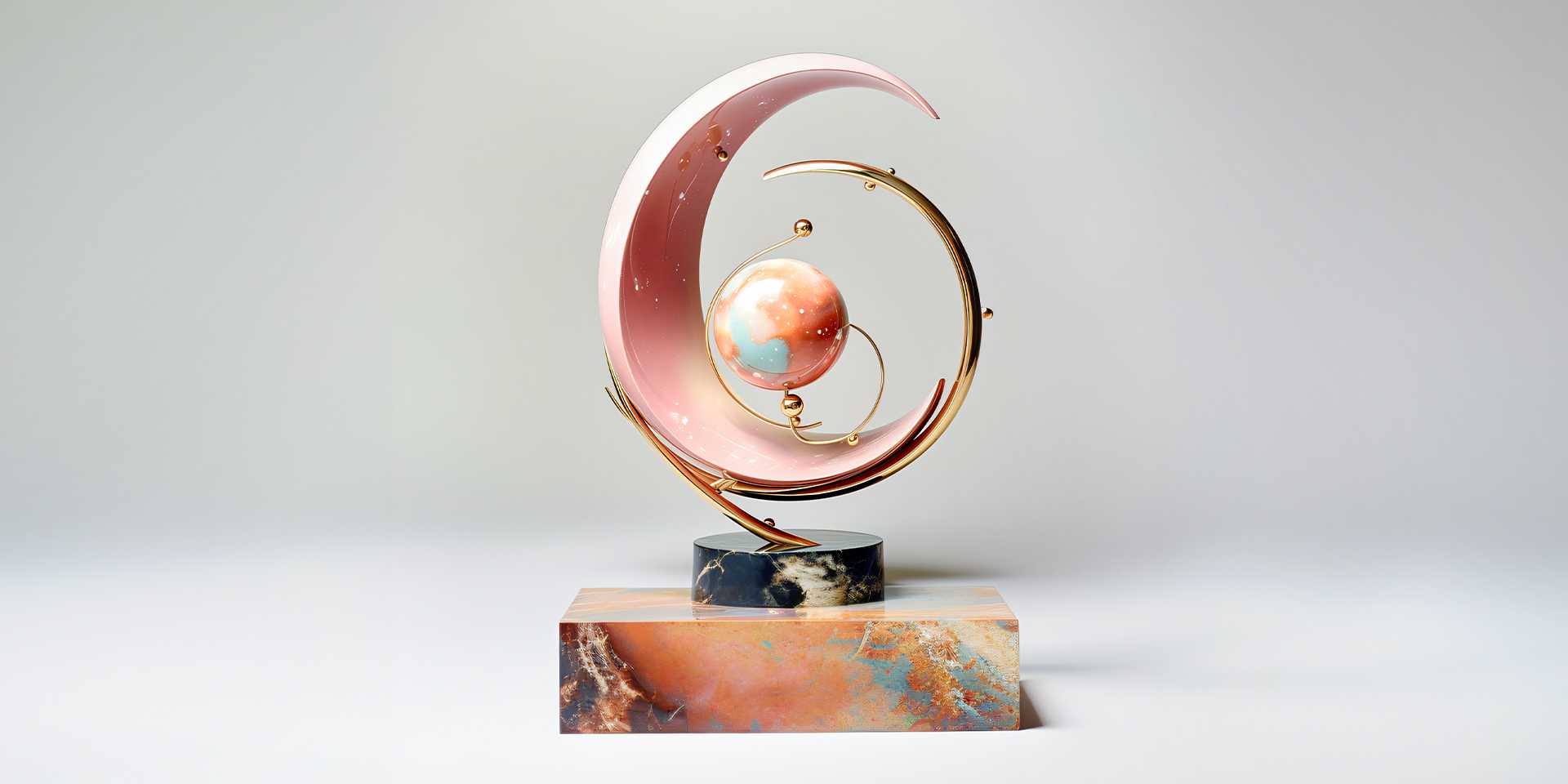
Without quantum mechanics, many of the technologies that we take for granted would be inconceivable. These include lasers, electron microscopes and atomic clocks, as well as medical imaging, electronics and semiconductors. It is difficult to think of another scientific theory that has been confirmed so frequently and so consistently since it was first elaborated 100 years ago. Of the four fundamental forces in physics that hold the world together on the atomic and subatomic level, three are based on concepts derived from quantum physics: electromagnetism, which is responsible for everyday phenomena such as light, electricity and magnetism; the strong nuclear force, which binds atoms together; and the weak nuclear force, which causes atomic nuclei to decay.
Yet the fourth fundamental force of physics, gravitation, which holds our universe together and explains the behaviour of planets, is notoriously hard to reconcile with quantum mechanics. This is because quantum mechanics is exceptionally good at explaining the interaction of particles at the atomic and subatomic level, but it rapidly runs into problems when tackling larger objects, which are subject to gravity. “Despite many attempts by luminaries such as Albert Einstein, Richard Feynman and Stephen Hawking, no one has yet been able to explain gravitation in terms of quantum physics,” explains Renato Renner, Professor for Theoretical Physics at ETH Zurich. Meanwhile, in the macroscopic world, Albert Einstein’s general theory of relativity continues to hold sway – another theory that, like quantum mechanics, has been confirmed by numerous experiments over the past 100 years. Without it, neither GPS devices nor watches would function accurately.
It might seem as if all this slots neatly into place in the world of physics – but, unfortunately, nothing could be further from the truth. The problem is that this description of the atomic and subatomic world doesn’t fit in with our picture of the cosmos. As Renner explains: “General relativity is incompatible with the principles of quantum mechanics.” For years now, physicists have been dreaming of combining the two theories to produce a unified picture of the physical world.
The curvature of space time
In 1915, Albert Einstein published his general theory of relativity, which rocked the established view of the physical world. Einstein departed from the received explanation of gravity as a force dependent on the mass of and distance between two planets, as formulated by Isaac Newton almost 200 years earlier. Instead, he introduced a new concept: space-time. “Einstein takes the familiar concept of three-dimensional space and fuses it with time to create a four-dimensional mathematical construct. He then explains gravity geometrically in terms of the curvature of space-time,” says Renner.
According to this schema, massive objects like planets create a dent in space-time. In turn, the geometry of this dent determines how objects move within the space-time continuum. In simple terms, space-time is like a trampoline upon which a heavy ball then creates a depression. If a tennis ball is placed at the edge of this depression, it will roll down towards the heavy ball. The concept of space-time serves to explain why, for example, clocks run faster on an airplane than back on Earth. Einstein’s theory was even able to explain why Mercury has such an eccentric orbit around the Sun.
Quantum fuzziness
Around the same time as Einstein, physicists such as Werner Heisenberg, Niels Bohr and Erwin Schrödinger were doing their best to upset our picture of the atomic and subatomic world. They posited that the realm where electrons, protons and other elementary particles are in constant motion, attracting and repelling one another, is subject to different laws. But whereas Einstein’s formulas can be used to calculate the orbit of the planets as precisely as if they were on rails, the objects of the quantum world cannot be described with the same precision. In the microcosm of atoms and particles, there are no such fixed orbits. There, the determinism of classical physics gives way to probabilistic statements. “An electron or proton can be in several places at the same time,” explains Professor Anna Soter, who conducts experimental research at the ETH Institute for Particle Physics and Astrophysics. “It is only through the act of measuring them that they acquire a determinate location. Beforehand, the best that we can do is to draw up a probability distribution.”
The general theory of relativity makes no provisions for such fuzziness. Were a particle to be in several places at one and the same time, it would no longer be possible to calculate the precise location where it makes a dent in space-time. Nevertheless, it is universally accepted that even the very smallest objects bend space-time and thus influence gravity. After all, they, too, are endowed with mass, just like larger objects. And since the earth is composed of particles, many physicists think it must be possible to incorporate general relativity into quantum mechanics. Yet, to this day, it remains unclear how gravitational force arises from these particles and their irregular movements.
Scientifica 2023
- Vom Versuch, das Grösste mit dem Kleinsten zu vereinen. external page Short lecture (in DE). R. Renner, Theor. Physics, ETH.
- Ein Tanz von Licht und Materie. external page Short lecture (in DE). Martin Frimmer, Photonics Laboratory, ETH.
- Mission Nano-MRI. external page Short lecture (in DE). Alexander Eichler, Laboratory for Solid State Physics, ETH.
- a computer made of light and sound particles. external page Exhibition stand. Hybrid Quantum Systems, ETH.
Gravity as information
Several theories have been proposed to explain gravitation on the basis of quantum physics. These include string theory and loop quantum gravity. A more recent approach, also being pursued at the chair of Professor Renner, is known as “It from Qubit”. This theory is based on the premise that the properties of space-time can be described in terms of entangled qubits – the basic unit of information in quantum computing. Unlike Einstein’s theory of relativity, this new approach is no longer purely geometrical in nature. Instead, it is based on the sum of qubit entanglements.
“Contrary to existing theories, ours isn’t concerned with what qubits are made of,” Renner explains. “What we’re interested in is how they are entangled with one another. These entangled qubits correspond to curved space-time in the macroscopic world.” For Renner, the search for quantum gravity is primarily about discovering the right patterns of entanglement between qubits. To illustrate this, we might think of space-time as the taut fabric on a trampoline, with each fibre representing a relation between qubits. An indication of how seriously this approach should be taken is that it delivers the same results as other theories when explaining theoretical quantities such as the entropy of black hole radiation.
New experiments
As Professor Soter explains, the problem today is not a shortage of grand theories but rather a lack of experiments that might provide insight into how gravity affects elementary particles. At present, there is no way of directly testing either It from Qubit or any other of the theories in this field.
Her research has therefore taken another tack. When physicists say that particles have mass and that they should therefore cause a curvature of space-time, they are assuming that the inertial mass of these particles corresponds to their gravitational mass. Inertial mass is a measure of an object’s resistance to a change in motion; gravitational mass is a measure of the force that gravity exerts upon an object. The assumption that there is no difference between the two is a key plank of general relativity and is known as the equivalence principle.
For Soter, however, this is by no means proven in the quantum world. “We can certainly measure the inertial mass of atoms, but no one has ever observed a falling particle that consists only of leptons and is therefore not affected by the strong interaction,” she explains. She hopes to change this with an experiment that aims to observe whether a horizontal beam of muonium atoms is subject to the pull of gravity and will therefore fall downwards like a jet of water from a garden hose.
If this turns out to be the case, it would be further proof of the need to unify quantum mechanics and general relativity. “But if we discover an anomaly here, and the muonium atoms do not fall as expected, then we have a big problem,” she says. This would create a minor sensation in the world of particle physics. Yet it would not be the first time an experiment had caused us to rethink our picture of the physical world.
About
Renato Renner is Professor for Theoretical Physics at the Department of Physics of ETH Zurich.
Anna Soter is Assistant Professor of Low Particle Physics at the Department of Physics of ETH Zurich.
Globe What holds the world together
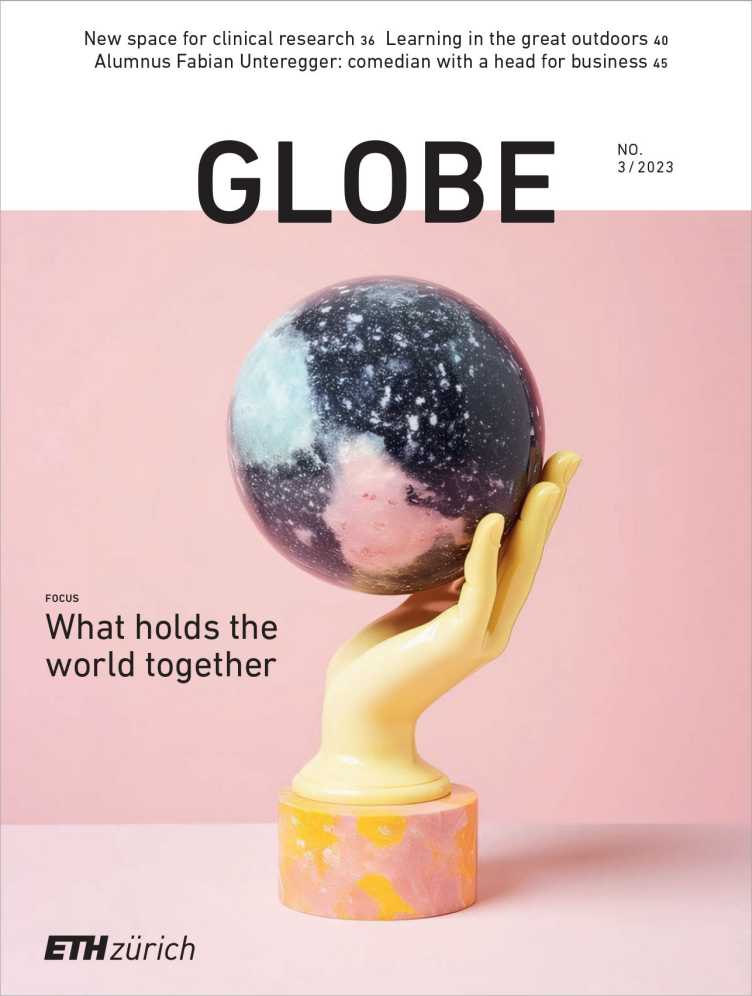
This text appeared in the 23/03 issue of the ETH magazine Globe.
Download Read whole issue (PDF, 4.6 MB)
Comments
No comments yet